I received my PhD in Entomology with a designated emphasis in Biology of Vector-Borne Diseases, from the University of California, Davis in 2008. I then joined Case Western Reserve University, Center for Global Health and Diseases, as a post-doctoral research associate (2009-2011) and then as a senior lecturer (2011-2013). During these four years I held a joint appointment as the head of Entomology at the Papua New Guinea Institute of Medical Research. I joined LSTM department of Vector Biology in February 2013, with a secondary appointment in the department of Parasitology.
Current research projects
Pleiotropic effects of insecticide resistance on vector behaviour and disease transmission.
Funded by Wellcome Trust
Insecticide resistance is the greatest threat to the control of deadly and debilitating vector-borne diseases such as malaria, Dengue and lymphatic filariasis. The scale-up of insecticide-based interventions over the last decade has led to the rapid emergence and spread of highly conserved resistance mechanisms across the globe. Insecticide resistance is recorded in all disease vectors worldwide, and resistance has evolved against all four classes of insecticides available for public health use. The current Zika epidemic emerged from a population of highly resistant Aedes aegypti in Recife, Brazil and the re-emergence of malaria has been attributed to resistant populations in some settings. While the factors that contribute to vector-borne disease epidemics are complex, the challenge of controlling disease in multi-resistant vector populations with limited insecticide-based tools is substantial.
In addition to increasing survivorship in the presence of insecticides, mechanisms of resistance influence many other aspects of disease transmission. Pleiotropic effects of insecticide resistance may include depletion of energetic resources, changes in immune function, biochemical imbalance in tissues necessary for parasite development and a compromised nerve system. As such, the reach of insecticide resistance is even greater than anticipated, also influencing vector competence (the intrinsic ability to develop and transmit infective stage pathogens) and vectorial capacity (the broader suite of environmental, behavioural and genetic traits that influence transmission).
It is crucial to understand the true impacts of resistance to improve disease pattern predictions, plan interventions and mitigate the effects of resistance on transmission. Are pleiotropic effects on vector physiology and behaviour also driving the spread of transmission? What can we expect as disease spreads through neighbouring vector populations with different resistance profiles?
This proposal aims to establish mosquito colonies which will enable further work on how resistance mechanisms influence mosquito behaviours, bionomics and immunity in an era where insecticide resistance is prevalent. The specific objectives include:
1) Establish and fully characterise strains of Aedes aegypti exhibiting the most dominant and widespread resistance mechanisms
2) Determine how multi-generational insecticide exposure and resistance reversal influence the transcription of genes related to resistance, detoxification, immunity and development.
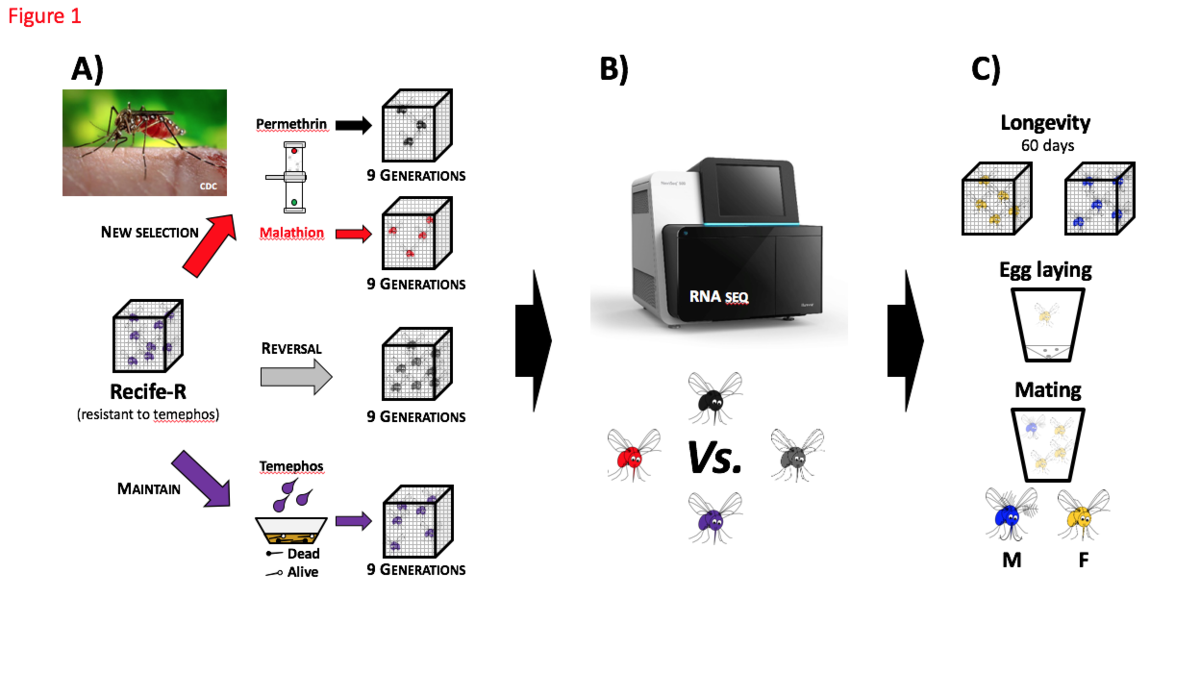
Figure 1 – Methods to evaluate the Pleiotropic effects of metabolic resistance in Aedes aegypti (Recife-R strain)
A: Establish four colony lines of Aedes aegypti from Recife-R strain with metabolic resistant to temephos (organophosphate). B: Sequencing all colony lines by RNA-seq (Illumina) for comparative analysis. C: Assays to determine fitness and ecological differences between these four colony lines.
Purple mosquito: Recife-R maintained under standard conditions, one temephos selection (0.5mg/L) at larvae stage every three generations; Black mosquito: Recife-R continuously selected with permethrin (pyrethroid) at adult stage; Red mosquito: Recife-R continuously selected with malathion (organophosphate) at adult stage; Grey mosquito: Recife-R maintained without insecticide pressure in order to reverse insecticide resistance. M: male mosquito; F: female mosquito.
Vector excreta surveillance to support the rapid detection of vector-borne diseases.
Funded by Medical Research Council
More than half of the world’s population is at risk of deadly and debilitating vector-borne diseases. These include neglected tropical diseases (NTDs) currently targeted for elimination such as lymphatic filariasis, onchocerciasis, leishmaniasis, human African trypanosomiasis and Chagas disease. They also include emerging threats which require active surveillance and rapid response such as the arboviral diseases Zika, dengue and chikungunya. Both eliminable and emerging vector-borne diseases require new, sustainable and low cost approaches to improve surveillance in order to protect vulnerable populations.
Xenomonitoring, the use of vectors to detect the presence of a pathogen in the human population, has been proposed as an essential approach to meet some of the challenges in vector borne disease surveillance. However, there are still significant limitations, which is why it is not used at scale in developing countries. Our group has adapted a novel approach to molecular xenomonitoring with promise to detect a range of vector-borne diseases in a community. It addresses many of the existing challenges and is well suited for integration across multiple disease control programmes. The vector excreta surveillance system (VESS) involves screening mosquito excreta and faeces (E/F) for parasites that have been ingested with an infected bloodmeal. The strengths of this approach over current community-wide surveillance strategies are:
- Non-invasive. VESS can be repeated frequently in at-risk communities because it requires minimal community participation
- Sampling the at-risk population. Mosquitoes will take blood from members of the community that are highly exposed, even those that are difficult to reach and do not readily participate in interventions and treatments.
- Capacity for large pool sizes. Mosquito excreta is a ‘clean’ sample with few inhibitors and we have yet to find a limit to detection in our experiments using E/F from up to 500 control mosquitoes.
- Works with existing mosquito collection. Mosquitoes are routinely collected in many countries as part of vector surveillance, for malaria or arboviruses. Mosquitoes can be collected in the usual way and the collection cups tested for a range of parasites and pathogens, without using the mosquito samples.
- No expertise needed. Where mosquito collection is not routine, VESS would not require any expertise in mosquito collection and identification. E/F can be collected from vectors and non-vectors alike using low cost or commercial mosquito traps.
- Adaptable. Our laboratory-based studies have shown that detection of nematode and protozoan DNA is possible in vectors and non-vectors. With further research this strategy may be adaptable to other vector-borne diseases, vector-borne zoonoses, and even blood borne pathogens that are incidentally ingested and excreted by mosquitoes.
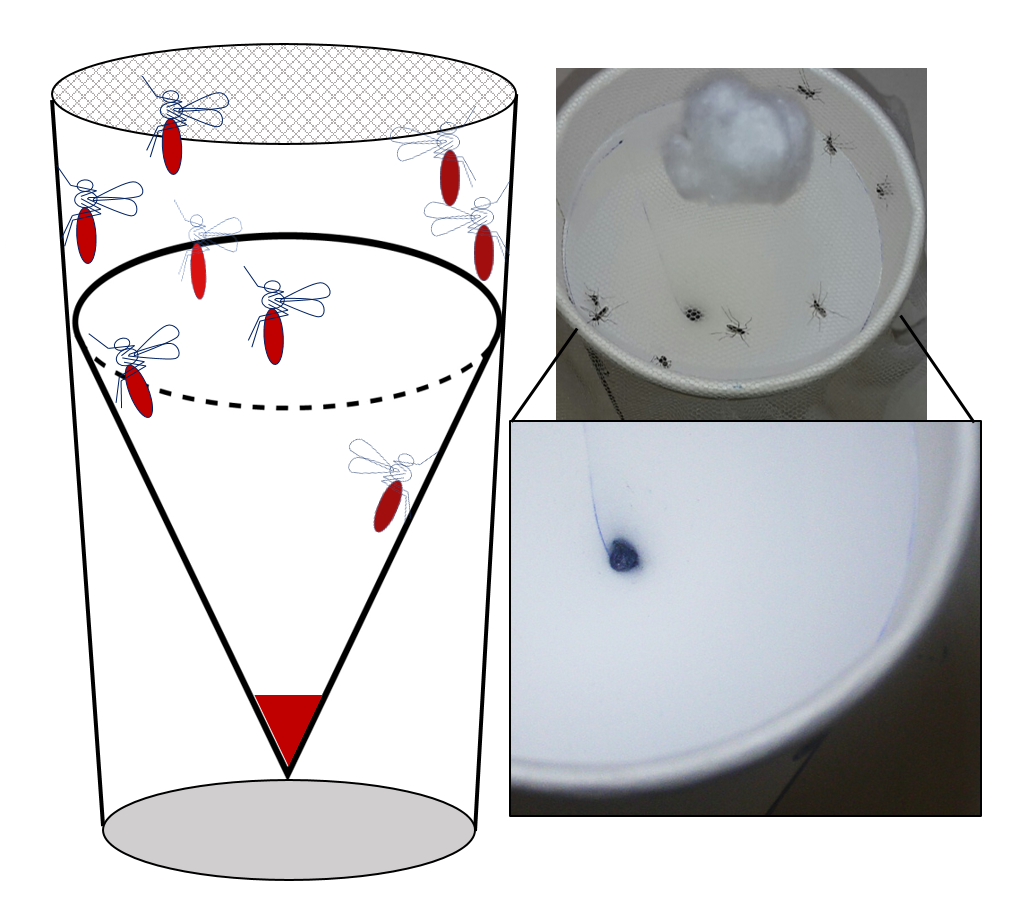
Figure 2. Netted mosquito collection cup with removable hydrophobic collection reservoir. Mosquitoes are provided with sugar water and E/F pools at the bottom for easy collection (left). Photo of resting mosquitoes that have been fed dyed sugar water with E/F deposition seen at the bottom with no residue on the walls (right).
Integrated complementary strategies to support the elimination of Lymphatic Filariasis in Ghana.
COUNTDOWN consortium, funded by the UK Department for International Development
It has become clear that achieving effective treatment coverage in mass drug administration (MDA) alone for neglected tropical diseases (NTDs) such as lymphatic filariasis (LF) is not enough to achieve the 2020 NTD targets. The World Health Organization recommends complementary strategies to accelerate transmission interruption, including improved vector control, the provision of sanitation and hygiene, health awareness and capacity building. As elimination becomes a target for LF, it is necessary to address the underlying causes allowing the disease to persist despite long periods of intervention. LF prevalence is highly heterogeneous and hotspots persist despite successful MDA programs. It is likely that these heterogeneities are driven by differences in vector characteristics such as biting density, vector competence, host-seeking behaviours and possibly host and vector genetic factors. As such, complementary strategies to reduce exposure are crucial to the success of the elimination programme. The LF elimination programme in Ghana has achieved tremendous success through the MDA campaign, and many regions have met the criteria to stop distribution. In these regions it will be crucial to sustain the programme gains and prevent resurgence. There is evidence that integration of vector control and MDA can accelerate elimination but although vector control is a part of many national malaria control programmes, coverage and compliance are sub-optimal. Also, long lasting insecticide-treated nets (LLINs) are not distributed along with MDA and there is no coordination between National Malaria Control Programme and LF elimination programme.
The overall aim of our work in Ghana study is to identify the characteristics of the remaining endemic regions and inform the integrated distribution of drugs and nets to stop transmission and achieve and sustain elimination. We are identifying the parasitological, entomological and epidemiological determinants of persistent LF in multiple endemic communities representative of different environments and ecologies in the Western and Northern Regions, and in collaboration with social scientists and health economists we are also looking at the social determinants and barriers to access and uptake of drugs during MDA and bed nets to improve health service delivery.
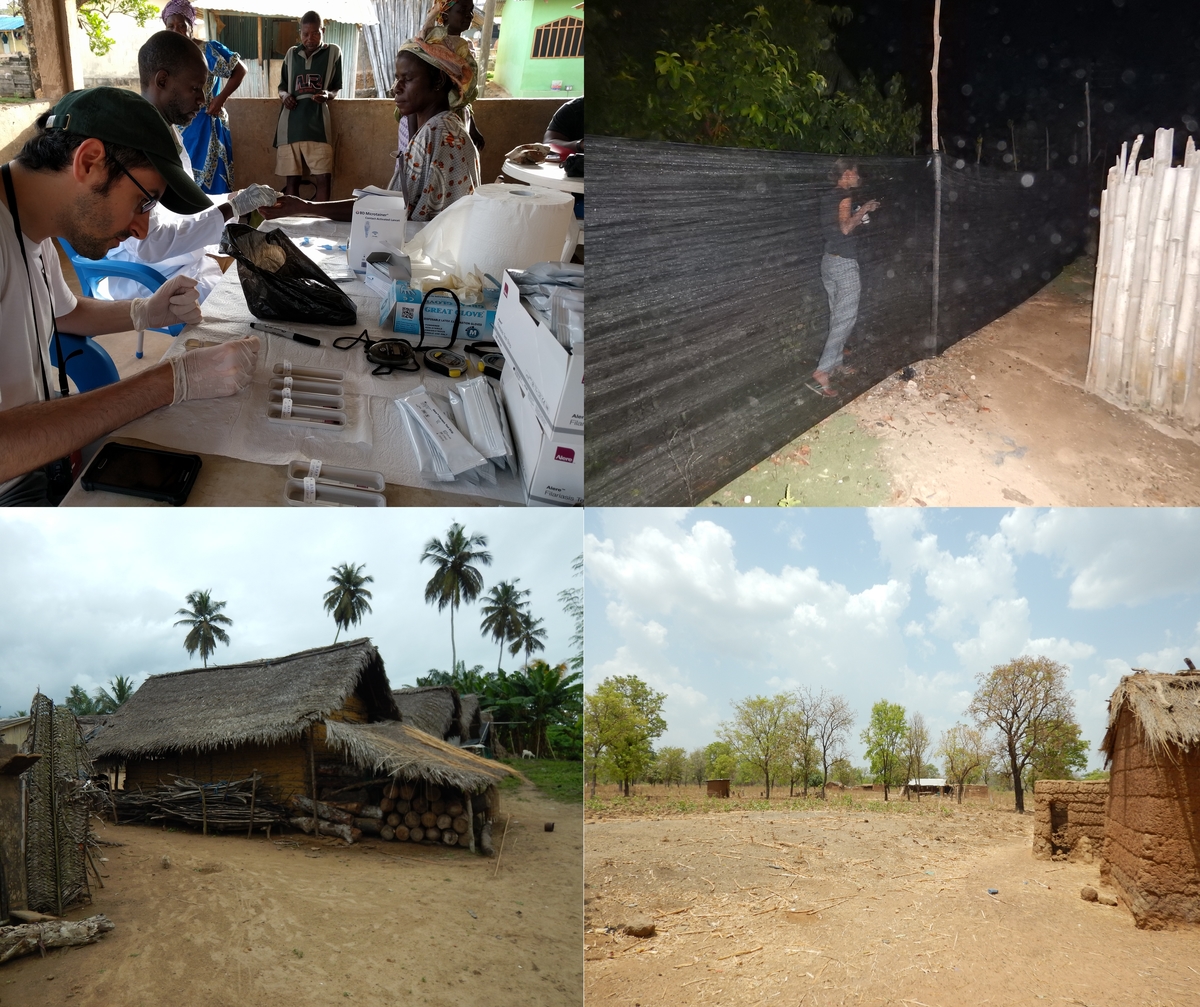
Figure 3.Testing community members for Wuchereria bancrofti antigen in blood (upper left); collecting mosquitoes entering and leaving the community using a barrier screen (upper right); community in the Western Region coast (lower left) and in the Northern Region savannah (lower right)
Teaching
Various modules for undergraduate and post-graduate courses including mosquito behaviour, mosquito biology, vector control, vector-parasite interactions, malaria transmission and filariasis transmission.
Lab members
Current and Past Students supervised
Emma Gordon, MSc Liverpool School of Tropical Medicine, major advisor 2016-2017
Millicent Opoku, MSc Liverpool School of Tropical Medicine, major advisor 2016-2017
Fiona Moon, MSc Liverpool School of Tropical Medicine, major advisor 2015
Mary Gray, BSc. Honours, University of Liverpool, major advisor 2015-2016
Thilini Weeraratne,PhD University of Peradeniya-Sri Lanka and LSTM, co-advisor, 2014 -
Clarer Jones, PhD Muhimbili University and LSTM, co-advisor, 2014 -
Dingani Chinula, MSc Liverpool School of Tropical Medicine, co-advisor, 2014
Justin Kumala, MSc Liverpool School of Tropical Medicine, major advisor, 2014
Katherine Gleave, MSc Liverpool School of Tropical Medicine, major advisor, 2014
David Edwards, BSc. Honours, University of Liverpool, major advisor, 2013-2014
Rebecca Vinit, BSc. Honours, University of Papua New Guinea, major advisor, 2012 –2013
John Keven, MSc Entomology, University of Michigan, committee member, 2011 – 2013
Gussy Koimbu, BSc. Honours, University of Papua New Guinea, major advisor, 2011 –2013
Melinda Susapu, PhD Liverpool School of Tropical Medicine, in-country supervisor, 2010 –
John Keven, BSc. Honors, University of Papua New Guinea, major advisor, 2009 – 2010